Hydrogen Gas in Cancer TreatmentScientific Research
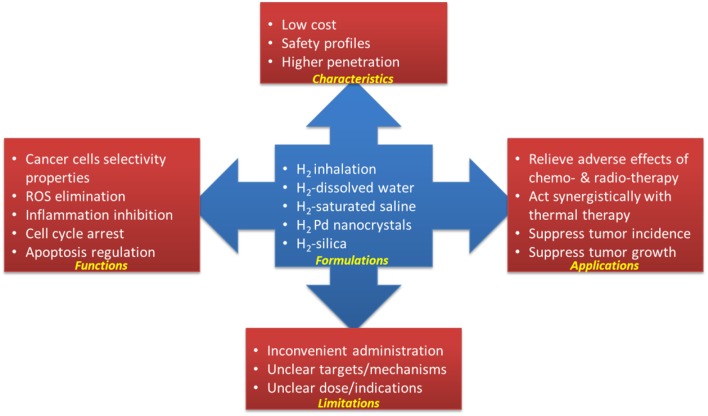
Hydrogen Gas in Cancer Treatment
Sai Li , 1, † Rongrong Liao , 2, † Xiaoyan Sheng , 2, † Xiaojun Luo , 3 Xin Zhang , 1 Xiaomin Wen , 3 Jin Zhou , 2, * and Kang Peng 1, 3, *
Gas signaling molecules (GSMs), composed of oxygen, carbon monoxide, nitric oxide, hydrogen sulfide, etc., play a crucial role in regulating signaling and cellular homeostasis. Interestingly, through various routes of administration, these molecules also showed potential to treat cancer. Recently, hydrogen gas (molecular formula: H2) has emerged as another GSM with various biological activities, including anti-inflammatory, anti-ROS and anti-cancer effects. Growing evidence suggests that hydrogen can both alleviate side effects caused by traditional chemotherapeutic drugs and inhibit the growth of cancer cells and xenograft tumors, suggesting its broad and effective use in clinical therapy. In the current review, we summarize these studies and discuss potential mechanisms. The application of hydrogen in cancer therapy is still in its infancy and requires further mechanistic studies and the development of portable instruments.
Introduction
Gas Signal Molecules (GSMs) refer to a group of gas molecules such as oxygen (1), nitric oxide (2), carbon monoxide (3), hydrogen sulfide (4), sulfur dioxide (5, 6), ethylene (7). , 8) etc. These gaseous molecules have several key functions in regulating cell biology in vivo through signal transduction (9). More importantly, when used directly or in certain pharmaceutical formulations, certain GSMs are useful as therapeutics for primary cancers as well as for multidrug-resistant cancers (9-13). In addition, some of these GSMs can be produced in the body by various bacteria or enzymes (eg, nitric oxide, hydrogen sulfide), suggesting that they are more compatible molecules that may have fewer side effects than traditional chemotherapy drugs (9, 14). , 15) . . Hydrogen has recently been recognized as another important GSM in biology, with tantalizing potential in healthcare due to its role in preventing cellular damage from various attacks
For the molecular formula H2, hydrogen is the lightest molecule in nature, making up only about 0.5 parts per million (ppm) of all gases. Hydrogen is essentially a colorless, odorless, tasteless, non-toxic, highly flammable gas that can form explosive mixtures with air at concentrations ranging from 4% to 74% and can be triggered by sparks, heat, or sunlight. Hydrogenases of certain members of the human gastrointestinal microbiota can generate small amounts of hydrogen gas from unabsorbed carbohydrates in the gut through catabolism and metabolism (20, 21), which are then partially diffused into the bloodstream and released and detected in vivo in exhalation of respiration (20), indicating its potential as a biomarker.
As the lightest molecule in nature, hydrogen exhibits attractive permeation properties as it can diffuse rapidly across cell membranes. Animal model studies have shown that after oral administration of ultra-hydrogen-rich water and ultra-hydrogen-rich saline intraperitoneally, the hydrogen concentration peaks at 5 minutes; while intravenous injection of HSRS takes 1 minute. Another in vivo study tested the distribution of hydrogen in the brain, liver, kidney, mesenteric fat, and thigh muscles of rats after inhalation of 3% hydrogen. The order of hydrogen concentration at saturation was liver, brain, mesentery, muscle, kidney, indicating that the distribution between organs in rats was different. With the exception of thigh muscles which take longer to reach saturation, other organs take 5-10 minutes to reach maximum hydrogen concentration. Meanwhile, the liver has the highest Cmax. This information may guide future clinical applications of hydrogen.
Although hydrogen gas was studied as early as 1975 as a treatment for mouse models of cutaneous squamous cell carcinoma, its potential for medical applications was not fully explored until 2007 by Oshawa et al. Hydrogen has attracted worldwide attention by being reported to ameliorate cerebral ischemia-reperfusion injury by selectively reducing cytotoxic reactive oxygen species including hydroxyl radicals and peroxynitrite. Through various formulations, hydrogen has been used as a drug for the treatment of Parkinson’s disease, rheumatoid arthritis, brain injury, ischemia-reperfusion injury, diabetes and other diseases.
What’s more, hydrogen has been shown to improve clinical endpoints and surrogate markers, from metabolic diseases to chronic systemic inflammatory diseases and cancer.A 2016 clinical study showed that hydrogen inhalation is safe for patients with post-cardiac arrest syndrome, and its further therapeutic use in other conditions becomes more attractive.
In the current overview, we focus on applications in cancer therapy. Generally, hydrogen can exert its biological functions by regulating ROS, inflammatory and apoptotic events.
Hydrogen Gas Selectively Scavenges Hydroxyl Radical and Peroxynitrite, and Regulates Certain Antioxidant Enzymes
To date, many studies have shown that hydrogen does not target specific proteins, but instead regulates several key players in cancer, including ROS and certain antioxidant enzymes (36).
ROS refers to a series of unstable oxygen-containing molecules, including singlet oxygen, hydrogen peroxide, hydroxyl radicals, superoxide, nitric oxide, and peroxynitrite. Once generated in vivo, ROS can damage proteins, DNA/RNA, and lipids in cells due to their high reactivity, causing severe damage, leading to apoptosis. The presence of ROS can induce cellular stress and damage through a mechanism called oxidative stress, leading to cell death. Normally, under bodily conditions, cells, including cancer cells, maintain a balance between the formation and elimination of ROS, which is critical for their survival. Excessive production of ROS, resulting from an imbalance of regulatory systems or external chemical attack, can trigger an internal cascade of apoptosis and cause severe toxic effects.
Hydrogen can act as a ROS regulator. First, as demonstrated by Ohsawa et al. Hydrogen was demonstrated to selectively scavenge the most cytotoxic ROS, •OH, as tested in a rat model of acute cerebral ischemia and reperfusion (26). Another study also confirmed that hydrogen can reduce oxygen toxicity induced by hyperbaric oxygen by effectively reducing •OH (46).
Second, hydrogen can induce the expression of some antioxidant enzymes that can eliminate ROS and play a key role in regulating redox homeostasis in cancer cells (42, 47). Studies have shown that hydrogen treatment increases the expression of superoxide dismutase (SOD) (48), heme oxygenase 1 (HO-1) (49), and nuclear factor erythroid 2-related factor 2 (Nrf2) (50). , significantly increased and enhanced its ROS-eliminating potential.
By modulating ROS, hydrogen can be used as an adjunctive regimen to reduce side effects in cancer therapy without reversing the cytotoxicity of other therapies such as radiotherapy and chemotherapy (48, 51). Interestingly, the administration of hydrogen can initially reduce ROS levels due to the excess production of ROS in cancer cells (38), but due to a compensatory effect, it triggers more ROS production, leading to cancer cell killing.
Hydrogen Gas Suppresses Inflammatory Cytokines
Inflammatory cytokines are a group of signaling molecules that mediate innate immune responses, and their dysregulation may lead to many diseases, including cancer (53-55). Typical inflammatory cytokines include interleukins (ILs) secreted by leukocytes, tumor necrosis factors (TNFs) secreted by macrophages, both of which have been shown to be closely associated with cancer initiation and progression (56-59), and Both ILs and TNFs can be inhibited by hydrogen gas (60, 61).
Inflammation induced by chemotherapy in cancer patients not only causes severe side effects (62, 63), but also leads to cancer metastasis and treatment failure (64, 65). By modulating inflammation, hydrogen can prevent tumor formation and progression, as well as reduce side effects from chemotherapy/radiotherapy (66).
Hydrogen Gas Inhibits/Induces Apoptosis
Apoptosis, also known as programmed cell death, can be triggered by extrinsic or intrinsic signals and proceeds through various molecular pathways, serving as an effective cancer treatment strategy (67, 68). In general, apoptosis can be induced by (1) activation of cell surface death receptors (such as Fas, TNF receptors, or TNF-related apoptosis-inducing ligands), (2) survival signals (such as epidermal growth factor receptor, pro- Mitogen-activated protein kinase or phosphoinositide 3-kinase) and (3) activation of pro-apoptotic B-cell lymphoma 2 (Bcl-2) family proteins or down-regulation of anti-apoptotic proteins (such as X-linked inhibitor of apoptosis protein , survival and der apoptosis inhibitors) (69, 70).
Hydrogen can regulate intracellular apoptosis by affecting the expression of apoptosis-related enzymes. At a certain concentration, it can both act as an inhibitor of apoptosis by inhibiting pro-apoptotic B-cell lymphoma-2-associated X protein (Bax), caspase-3, 8, 12 and anti-apoptotic B-cell-enhancing lymphoma. Action-2 (Bcl-2) (71) or by contrasting mechanisms as an inducer of apoptosis (72), suggesting its potential to protect normal cells from anticancer drugs or inhibit cancer cells.
Hydrogen Gas Exhibits Potential in Cancer Treatment
Hydrogen Gas Relieves the Adverse Effects Related to Chemotherapy/Radiotherapy
Chemotherapy and radiotherapy remain the main strategies for treating cancer (73, 74). However, cancer patients receiving these treatments often experience fatigue and reduced quality of life (75-77). The spike in ROS formation during treatment is thought to cause side effects, leading to significant oxidative stress and inflammation (41, 42, 78). Therefore, hydrogen gas benefits from its antioxidant, anti-inflammatory and other cytoprotective properties and can be used as an adjunctive treatment regimen to suppress these side effects.
Patients with non-small cell lung cancer frequently develop severe acute interstitial pneumonia when treated with gefitinib, an epidermal growth factor receptor inhibitor (79). In a mouse model of oral gefitinib and intraperitoneal injection of naphthalene, hydrogen-rich water treatment resulted in a significant reduction of inflammatory cytokines such as IL-6 and TNFα due to severe lung injury due to oxidative stress. Bronchoalveolar lavage fluid relieves pneumonia. More importantly, hydrogen-rich water did not affect the overall antitumor effect of gefitinib in vitro and in vivo, on the contrary, it counteracted the weight loss induced by gefitinib and naphthalene and improved overall survival, suggesting that hydrogen As a promising adjuvant, it has the potential to be used in clinical practice to improve the quality of life of cancer patients (80).
Doxorubicin, an anthracycline antibiotic, is a potent anticancer agent for various cancers, but its use is limited to fatal dilated cardiomyopathy and hepatotoxicity (81, 82). An in vivo study showed that intraperitoneal injection of hydrogen-rich saline improved doxorubicin-induced mortality and cardiac insufficiency. This treatment also attenuated histopathological changes in rat serum such as B. brain natriuretic peptide (BNP), aspartate aminotransferase (AST), alanine aminotransferase (ALT), albumin and malondialdehyde (MDA) levels in serum.Mechanistically, hydrogen-rich saline significantly reduced ROS levels and inflammatory cytokines TNF-α, IL-1β, and IL-6 in cardiac and liver tissues. Hydrogen-rich saline also induced a decrease in the expression of apoptotic Bax, cleaved caspase-3, and higher anti-apoptotic Bcl-2, resulting in decreased apoptosis in both tissues (71). The present study suggests that hydrogen-rich saline treatment may enhance its protective effect by inhibiting the inflammatory TNF-α/IL-6 signaling pathway, increasing cleaved C8 expression and Bcl-2/Bax ratio, and attenuating apoptosis. Heart and liver tissue (71).
Hydrogen-rich water also showed renoprotective effects against cisplatin-induced nephrotoxicity in rats. In the study, blood oxygen content-dependent (BOLD) magnetic resonance imaging (MRI) images obtained in the different treatment groups showed creatinine and blood urea nitrogen (BUN) levels, two parameters associated with nephrotoxicity were significantly higher in the cisplatin-treated group to those in the control group. Treatment with hydrogen-rich water significantly reversed the toxic effects and showed higher transverse relaxation rates by eliminating oxygen radicals (83, 84).
Another study showed that both inhaling hydrogen (1% hydrogen in air) and drinking hydrogen-rich water (0.8 mM hydrogen in water) reversed cisplatin-induced mortality and weight loss due to its antioxidant properties . Both treatments improved metamorphosis, while reducing renal apoptosis and nephrotoxicity, as assessed by serum creatinine and BUN levels. More importantly, hydrogen did not affect the antitumor activity of cisplatin against cancer cell lines in vitro and in tumor-bearing mice (85). Similar results were found by Meng et al. observed because they showed that hydrogen-rich saline inhibited the release of follicle-stimulating hormone, increased estrogen levels, improved follicular development and reduced cisplatin-induced damage to the ovarian cortex. In this study, treatment with cisplatin resulted in higher levels of oxidative products and inhibition of antioxidant enzyme activity.Hydrogen-rich saline can reverse these toxic effects by reducing MDA and restoring the activity of two important antioxidant enzymes, superoxide dismutase (SOD) and catalase (CAT). Furthermore, hydrogen-rich saline stimulates the Nrf2 pathway in ovarian-damaged rats (86).
The mFOLFOX6 regimen, consisting of folinic acid, 5-fluorouracil, and oxaliplatin, is used as first-line treatment for metastatic colorectal cancer, but it also has toxic effects on the liver, resulting in poor quality of life in patients (87, 88). . in China A clinical study was conducted to investigate the protective effect of hydrogen-rich water on liver function in colorectal cancer patients treated with mFOLFOX6 chemotherapy (144 patients, 136 of whom were included in the final analysis). The results showed that the placebo group had mFOLFOX6 chemotherapy-induced adverse reactions, as measured by increased levels of ALT, AST, and indirect bilirubin (IBIL), while the hydrogen-rich water combination group had no difference in liver function during treatment, possibly due to it. antioxidant activity, suggesting that it is a promising protective agent for ameliorating mFOLFOX6-induced liver injury
Most of the adverse effects of ionizing radiation on normal cells are caused by hydroxyl radicals. Combining radiation therapy with some forms of hydrogen may help alleviate these side effects (89). In fact, several studies have found that hydrogen can protect cells and mice from radiation (48, 90).
Tested in a rat skin injury model using a 44 Gy electron beam, the hydrogen-rich water-treated group had a higher proportion of SOD activity and less MDA and IL-6 in the injured tissue compared to the control group and distilled water. water group. In addition, hydrogen-rich water shortens the healing time and improves the healing rate of skin lesions (48).
Gastrointestinal toxicity is a common side effect induced by radiotherapy, which impairs the life quality of cancer patients (91). As shown in Xiao et al.’s study in mice model, hydrogen-water administration via oral gavage increased the survival rate and body weight of mice which were exposed to total abdominal irradiation, accompanied with an improvement in gastrointestinal tract function and the epithelial integrity of the small intestine. Further microarray analysis revealed that hydrogen-water treatment up-regulated miR-1968-5p, which then up-regulated its target myeloid differentiation primary response gene 88 (MyD88, a mediator in immunopathology, and gut microbiota dynamics of certain intestinal diseases involving toll-like receptors 9) expression in the small intestine after total abdominal irradiation (92).
Gastrointestinal toxicity is a common side effect of radiation therapy and affects the quality of life of cancer patients (91). As reported in the study by Xiao et al. It was demonstrated in a mouse model that administration of hydrogen water by oral gavage increased survival and body weight in mice exposed to whole abdominal irradiation, accompanied by improvements in gastrointestinal function and intestinal epithelial integrity. Further microarray analysis revealed that hydrogen water treatment up-regulated miR-1968-5p and then mutated its primary myeloid differentiation response gene 88 (MyD88, a mediator of immunopathology, and gut microbiota dynamics in certain intestinal diseases). Charges and recipients 9) in the small intestine after whole abdominal irradiation (92).
Hydrogen Gas Acts Synergistically With Thermal Therapy
A recent study found that hydrogen can enhance the effects of photothermal therapy. Zhao et al. Hydrated Pd nanocrystals (named PdH0.2) were designed as multifunctional hydrogen carriers for tumor-directed delivery (due to 30 nm cubic Pd nanocrystals) and controlled release of bioreductive hydrogen (due to lattice-bound hydrogen). PD). As shown in this study, hydrogen evolution can be modulated by the power and duration of near-infrared (NIR) irradiation. Treatment with PdH0.2 nanocrystals plus NIR irradiation resulted in higher initial ROS loss in cancer cells, and the subsequent ROS rebound was also much higher than in normal cells, resulting in more apoptosis and severe inhibition of mitochondrial metabolism in cancer cells, but abnormal cells. The combination of PdH0.2 nanocrystals and NIR irradiation greatly enhanced the anticancer efficacy of hyperthermia and achieved a synergistic anticancer effect. The in vivo safety assessment showed that the injection dose of 10 mg kg-1 PdH0.2 nanocrystals did not cause death, and there were no changes in many blood indicators, and no damage to liver and kidney function. In a 4T1 mouse breast cancer tumor model and a B16-F10 melanoma model, the combined treatment of PdH0.2 nanocrystals and NIR irradiation showed synergistic anticancer effects with significant tumor suppressive effects compared with hyperthermia. Meanwhile, the combination group showed no significant damage to the heart, liver, spleen, lung, and kidney, indicating appropriate tissue safety and tolerability (52).
Hydrogen Gas Suppresses Tumor Formation
Reader. Drinking hydrogen-rich water has been reported to ameliorate renal injury induced by iron nitrilotriacetate (Fe-NTA) in rats, as evidenced by decreased serum creatinine and BUN levels. Hydrogen-rich water inhibited Fe-NTA-induced oxidative stress by reducing lipid peroxidation ONOO-, inhibiting the activities of NADPH oxidase and xanthine oxidase, up-regulating antioxidant catalase, and restoring renal mitochondrial function. Therefore, hydrogen treatment significantly attenuated Fe-NTA-induced inflammatory cytokines such as NF-κB, IL-6, and monocyte chemoattractant protein 1. More importantly, drinking hydrogen-rich water inhibited the expression of several cancer-related proteins, including vascular endothelial growth factor, transcription-3-phosphorylation signal converter and activator, and proliferating nuclear antigen in rats, thereby reducing renal incidence of cancer. Cell carcinomas and their inhibition lead to tumor growth. This work demonstrates that hydrogen-rich water is a promising regimen to mitigate Fe-NTA-induced renal injury and suppress early tumor events.
Nonalcoholic steatohepatitis (NASH), caused by oxidative stress from various stimuli, is one of the causes of liver cancer (94, 95). In a mouse model, administration of hydrogen-rich water decreased hepatic cholesterol and peroxisome proliferator-activated receptor-alpha (PPARα) expression and increased hepatic antioxidant activity compared with control and pioglitazone-treated groups role (96). Hydrogen-rich water showed potent inhibitory effects on inflammatory cytokines TNF-α and IL-6, oxidative stress and apoptosis biomarkers. As shown in the NASH-related hepatocarcinogenesis model, the hydrogen-rich water-treated group had a lower tumor incidence and smaller tumor volume than the control and pioglitazone-treated groups. The above results suggest that hydrogen-rich water has the potential to protect the liver and treat liver cancer (96).
Hydrogen Gas Suppresses Tumor Growth
In addition to being used as an adjuvant therapy, hydrogen can also inhibit the growth of tumors and tumor cells.
As reported in the study by Wang et al. As demonstrated on lung cancer cell lines A549 and H1975 cells, hydrogen gas inhibits cell proliferation, migration and invasion and induces significant apoptosis, such as CCK-8, wound healing, transwell assays and flow cytometry tests. Hydrogen prevents the cell cycle in the G2/M phase of both cell lines by inhibiting the expression of several cell cycle regulatory proteins, including cyclin D1, CDK4, and CDK6. Chromosome 3 (SMC3), a complex required for chromosome condensation in the cell cycle (97), is inhibited by hydrogen through ubiquitination. Importantly, an in vivo study showed that hydrogen treatment significantly inhibited tumor growth, as well as the expression of Ki-67, VEGF and SMC3. These data suggest that hydrogen could be used as a novel approach to the treatment of lung cancer (98).
Due to its physicochemical properties, the use of hydrogen in hospitals, medical institutions and laboratories is severely restricted. Reader. A solidified hydrogen-absorbing (H2) silica was designed to stably release molecular hydrogen into the cell culture medium. H2-silicic acid may inhibit cell viability in human esophageal squamous cell carcinoma (KYSE-70) in a concentration-dependent manner, whereas it requires higher doses to inhibit normal human esophageal epithelial cells (HEEpiCs), indicating its selectivity . This effect was further confirmed by apoptosis and cell migration assays in these two cell lines. Mechanistic studies suggest that H2-silicic acid exerts its anticancer effects by inducing H2O2 accumulation, cell cycle arrest, and mitochondrial pathway-mediated induction of apoptosis (72).
Hydrogen has recently been found to inhibit cancer stem cells (CSCs). Hydrogen reduces colony formation and spheroidization in human ovarian cancer cell lines Hs38.T and PA-1 cells by inhibiting the proliferation marker Ki67, the stem cell marker CD34, and angiogenesis. Hydrogen treatment significantly inhibited the proliferation, invasion and migration of Hs38.T and PA-1 cells. More importantly, hydrogen inhalation significantly suppressed tumor volume, as shown in the Hs38.T xenograft BALB/c nude mouse model (99).
Another recent study also demonstrated the role of hydrogen in inhibiting glioblastoma (GBM), the most common malignant brain tumor. In vitro studies have shown that hydrogen gas inhibits several markers associated with stem formation, thereby inhibiting glioma cell spheroidization, cell migration, invasion, and colony formation. In a rat orthotopic glioma model, twice-daily inhalation of hydrogen gas (67%) for 1 hour significantly inhibited GBM growth and improved survival, suggesting that hydrogen gas may be a promising drug for GBM treatment (100).
Discussion
What’s more, in most research papers, hydrogen is safe for cancer cells and some selectivity for normal cells, which is critical for clinical trials. A clinical study is underway in China to study the role of hydrogen in cancer recovery.
So far, several methods of administration have proven available and convenient, including inhalation, drinking hydrogen-dissolved water, injecting hydrogen-saturated saline, and performing a hydrogen bath. Hydrogen-rich water is non-toxic, inexpensive, easy to administer, and can easily diffuse into tissues and cells that cross the blood-brain barrier, suggesting its potential in the treatment of brain tumors. More well-structured and sufficiently secure wearables are needed.
References
DOI: 10.3389
Published on: 20190608
Hydrogen Gas in Cancer Treatment
Sai Li , 1, † Rongrong Liao , 2, † Xiaoyan Sheng , 2, † Xiaojun Luo , 3 Xin Zhang , 1 Xiaomin Wen , 3 Jin Zhou , 2, * and Kang Peng 1, 3, *
Gas signaling molecules (GSMs), composed of oxygen, carbon monoxide, nitric oxide, hydrogen sulfide, etc., play a crucial role in regulating signaling and cellular homeostasis. Interestingly, through various routes of administration, these molecules also showed potential to treat cancer. Recently, hydrogen gas (molecular formula: H2) has emerged as another GSM with various biological activities, including anti-inflammatory, anti-ROS and anti-cancer effects. Growing evidence suggests that hydrogen can both alleviate side effects caused by traditional chemotherapeutic drugs and inhibit the growth of cancer cells and xenograft tumors, suggesting its broad and effective use in clinical therapy. In the current review, we summarize these studies and discuss potential mechanisms. The application of hydrogen in cancer therapy is still in its infancy and requires further mechanistic studies and the development of portable instruments.
Introduction
Gas Signal Molecules (GSMs) refer to a group of gas molecules such as oxygen (1), nitric oxide (2), carbon monoxide (3), hydrogen sulfide (4), sulfur dioxide (5, 6), ethylene (7). , 8) etc. These gaseous molecules have several key functions in regulating cell biology in vivo through signal transduction (9). More importantly, when used directly or in certain pharmaceutical formulations, certain GSMs are useful as therapeutics for primary cancers as well as for multidrug-resistant cancers (9-13). In addition, some of these GSMs can be produced in the body by various bacteria or enzymes (eg, nitric oxide, hydrogen sulfide), suggesting that they are more compatible molecules that may have fewer side effects than traditional chemotherapy drugs (9, 14). , 15) . . Hydrogen has recently been recognized as another important GSM in biology, with tantalizing potential in healthcare due to its role in preventing cellular damage from various attacks
For the molecular formula H2, hydrogen is the lightest molecule in nature, making up only about 0.5 parts per million (ppm) of all gases. Hydrogen is essentially a colorless, odorless, tasteless, non-toxic, highly flammable gas that can form explosive mixtures with air at concentrations ranging from 4% to 74% and can be triggered by sparks, heat, or sunlight. Hydrogenases of certain members of the human gastrointestinal microbiota can generate small amounts of hydrogen gas from unabsorbed carbohydrates in the gut through catabolism and metabolism (20, 21), which are then partially diffused into the bloodstream and released and detected in vivo in exhalation of respiration (20), indicating its potential as a biomarker.
As the lightest molecule in nature, hydrogen exhibits attractive permeation properties as it can diffuse rapidly across cell membranes. Animal model studies have shown that after oral administration of ultra-hydrogen-rich water and ultra-hydrogen-rich saline intraperitoneally, the hydrogen concentration peaks at 5 minutes; while intravenous injection of HSRS takes 1 minute. Another in vivo study tested the distribution of hydrogen in the brain, liver, kidney, mesenteric fat, and thigh muscles of rats after inhalation of 3% hydrogen. The order of hydrogen concentration at saturation was liver, brain, mesentery, muscle, kidney, indicating that the distribution between organs in rats was different. With the exception of thigh muscles which take longer to reach saturation, other organs take 5-10 minutes to reach maximum hydrogen concentration. Meanwhile, the liver has the highest Cmax. This information may guide future clinical applications of hydrogen.
Although hydrogen gas was studied as early as 1975 as a treatment for mouse models of cutaneous squamous cell carcinoma, its potential for medical applications was not fully explored until 2007 by Oshawa et al. Hydrogen has attracted worldwide attention by being reported to ameliorate cerebral ischemia-reperfusion injury by selectively reducing cytotoxic reactive oxygen species including hydroxyl radicals and peroxynitrite. Through various formulations, hydrogen has been used as a drug for the treatment of Parkinson’s disease, rheumatoid arthritis, brain injury, ischemia-reperfusion injury, diabetes and other diseases.
What’s more, hydrogen has been shown to improve clinical endpoints and surrogate markers, from metabolic diseases to chronic systemic inflammatory diseases and cancer.A 2016 clinical study showed that hydrogen inhalation is safe for patients with post-cardiac arrest syndrome, and its further therapeutic use in other conditions becomes more attractive.
In the current overview, we focus on applications in cancer therapy. Generally, hydrogen can exert its biological functions by regulating ROS, inflammatory and apoptotic events.
Hydrogen Gas Selectively Scavenges Hydroxyl Radical and Peroxynitrite, and Regulates Certain Antioxidant Enzymes
To date, many studies have shown that hydrogen does not target specific proteins, but instead regulates several key players in cancer, including ROS and certain antioxidant enzymes (36).
ROS refers to a series of unstable oxygen-containing molecules, including singlet oxygen, hydrogen peroxide, hydroxyl radicals, superoxide, nitric oxide, and peroxynitrite. Once generated in vivo, ROS can damage proteins, DNA/RNA, and lipids in cells due to their high reactivity, causing severe damage, leading to apoptosis. The presence of ROS can induce cellular stress and damage through a mechanism called oxidative stress, leading to cell death. Normally, under bodily conditions, cells, including cancer cells, maintain a balance between the formation and elimination of ROS, which is critical for their survival. Excessive production of ROS, resulting from an imbalance of regulatory systems or external chemical attack, can trigger an internal cascade of apoptosis and cause severe toxic effects.
Hydrogen can act as a ROS regulator. First, as demonstrated by Ohsawa et al. Hydrogen was demonstrated to selectively scavenge the most cytotoxic ROS, •OH, as tested in a rat model of acute cerebral ischemia and reperfusion (26). Another study also confirmed that hydrogen can reduce oxygen toxicity induced by hyperbaric oxygen by effectively reducing •OH (46).
Second, hydrogen can induce the expression of some antioxidant enzymes that can eliminate ROS and play a key role in regulating redox homeostasis in cancer cells (42, 47). Studies have shown that hydrogen treatment increases the expression of superoxide dismutase (SOD) (48), heme oxygenase 1 (HO-1) (49), and nuclear factor erythroid 2-related factor 2 (Nrf2) (50). , significantly increased and enhanced its ROS-eliminating potential.
By modulating ROS, hydrogen can be used as an adjunctive regimen to reduce side effects in cancer therapy without reversing the cytotoxicity of other therapies such as radiotherapy and chemotherapy (48, 51). Interestingly, the administration of hydrogen can initially reduce ROS levels due to the excess production of ROS in cancer cells (38), but due to a compensatory effect, it triggers more ROS production, leading to cancer cell killing.
Hydrogen Gas Suppresses Inflammatory Cytokines
Inflammatory cytokines are a group of signaling molecules that mediate innate immune responses, and their dysregulation may lead to many diseases, including cancer (53-55). Typical inflammatory cytokines include interleukins (ILs) secreted by leukocytes, tumor necrosis factors (TNFs) secreted by macrophages, both of which have been shown to be closely associated with cancer initiation and progression (56-59), and Both ILs and TNFs can be inhibited by hydrogen gas (60, 61).
Inflammation induced by chemotherapy in cancer patients not only causes severe side effects (62, 63), but also leads to cancer metastasis and treatment failure (64, 65). By modulating inflammation, hydrogen can prevent tumor formation and progression, as well as reduce side effects from chemotherapy/radiotherapy (66).
Hydrogen Gas Inhibits/Induces Apoptosis
Apoptosis, also known as programmed cell death, can be triggered by extrinsic or intrinsic signals and proceeds through various molecular pathways, serving as an effective cancer treatment strategy (67, 68). In general, apoptosis can be induced by (1) activation of cell surface death receptors (such as Fas, TNF receptors, or TNF-related apoptosis-inducing ligands), (2) survival signals (such as epidermal growth factor receptor, pro- Mitogen-activated protein kinase or phosphoinositide 3-kinase) and (3) activation of pro-apoptotic B-cell lymphoma 2 (Bcl-2) family proteins or down-regulation of anti-apoptotic proteins (such as X-linked inhibitor of apoptosis protein , survival and der apoptosis inhibitors) (69, 70).
Hydrogen can regulate intracellular apoptosis by affecting the expression of apoptosis-related enzymes. At a certain concentration, it can both act as an inhibitor of apoptosis by inhibiting pro-apoptotic B-cell lymphoma-2-associated X protein (Bax), caspase-3, 8, 12 and anti-apoptotic B-cell-enhancing lymphoma. Action-2 (Bcl-2) (71) or by contrasting mechanisms as an inducer of apoptosis (72), suggesting its potential to protect normal cells from anticancer drugs or inhibit cancer cells.
Hydrogen Gas Exhibits Potential in Cancer Treatment
Hydrogen Gas Relieves the Adverse Effects Related to Chemotherapy/Radiotherapy
Chemotherapy and radiotherapy remain the main strategies for treating cancer (73, 74). However, cancer patients receiving these treatments often experience fatigue and reduced quality of life (75-77). The spike in ROS formation during treatment is thought to cause side effects, leading to significant oxidative stress and inflammation (41, 42, 78). Therefore, hydrogen gas benefits from its antioxidant, anti-inflammatory and other cytoprotective properties and can be used as an adjunctive treatment regimen to suppress these side effects.
Patients with non-small cell lung cancer frequently develop severe acute interstitial pneumonia when treated with gefitinib, an epidermal growth factor receptor inhibitor (79). In a mouse model of oral gefitinib and intraperitoneal injection of naphthalene, hydrogen-rich water treatment resulted in a significant reduction of inflammatory cytokines such as IL-6 and TNFα due to severe lung injury due to oxidative stress. Bronchoalveolar lavage fluid relieves pneumonia. More importantly, hydrogen-rich water did not affect the overall antitumor effect of gefitinib in vitro and in vivo, on the contrary, it counteracted the weight loss induced by gefitinib and naphthalene and improved overall survival, suggesting that hydrogen As a promising adjuvant, it has the potential to be used in clinical practice to improve the quality of life of cancer patients (80).
Doxorubicin, an anthracycline antibiotic, is a potent anticancer agent for various cancers, but its use is limited to fatal dilated cardiomyopathy and hepatotoxicity (81, 82). An in vivo study showed that intraperitoneal injection of hydrogen-rich saline improved doxorubicin-induced mortality and cardiac insufficiency. This treatment also attenuated histopathological changes in rat serum such as B. brain natriuretic peptide (BNP), aspartate aminotransferase (AST), alanine aminotransferase (ALT), albumin and malondialdehyde (MDA) levels in serum.Mechanistically, hydrogen-rich saline significantly reduced ROS levels and inflammatory cytokines TNF-α, IL-1β, and IL-6 in cardiac and liver tissues. Hydrogen-rich saline also induced a decrease in the expression of apoptotic Bax, cleaved caspase-3, and higher anti-apoptotic Bcl-2, resulting in decreased apoptosis in both tissues (71). The present study suggests that hydrogen-rich saline treatment may enhance its protective effect by inhibiting the inflammatory TNF-α/IL-6 signaling pathway, increasing cleaved C8 expression and Bcl-2/Bax ratio, and attenuating apoptosis. Heart and liver tissue (71).
Hydrogen-rich water also showed renoprotective effects against cisplatin-induced nephrotoxicity in rats. In the study, blood oxygen content-dependent (BOLD) magnetic resonance imaging (MRI) images obtained in the different treatment groups showed creatinine and blood urea nitrogen (BUN) levels, two parameters associated with nephrotoxicity were significantly higher in the cisplatin-treated group to those in the control group. Treatment with hydrogen-rich water significantly reversed the toxic effects and showed higher transverse relaxation rates by eliminating oxygen radicals (83, 84).
Another study showed that both inhaling hydrogen (1% hydrogen in air) and drinking hydrogen-rich water (0.8 mM hydrogen in water) reversed cisplatin-induced mortality and weight loss due to its antioxidant properties . Both treatments improved metamorphosis, while reducing renal apoptosis and nephrotoxicity, as assessed by serum creatinine and BUN levels. More importantly, hydrogen did not affect the antitumor activity of cisplatin against cancer cell lines in vitro and in tumor-bearing mice (85). Similar results were found by Meng et al. observed because they showed that hydrogen-rich saline inhibited the release of follicle-stimulating hormone, increased estrogen levels, improved follicular development and reduced cisplatin-induced damage to the ovarian cortex. In this study, treatment with cisplatin resulted in higher levels of oxidative products and inhibition of antioxidant enzyme activity.Hydrogen-rich saline can reverse these toxic effects by reducing MDA and restoring the activity of two important antioxidant enzymes, superoxide dismutase (SOD) and catalase (CAT). Furthermore, hydrogen-rich saline stimulates the Nrf2 pathway in ovarian-damaged rats (86).
The mFOLFOX6 regimen, consisting of folinic acid, 5-fluorouracil, and oxaliplatin, is used as first-line treatment for metastatic colorectal cancer, but it also has toxic effects on the liver, resulting in poor quality of life in patients (87, 88). . in China A clinical study was conducted to investigate the protective effect of hydrogen-rich water on liver function in colorectal cancer patients treated with mFOLFOX6 chemotherapy (144 patients, 136 of whom were included in the final analysis). The results showed that the placebo group had mFOLFOX6 chemotherapy-induced adverse reactions, as measured by increased levels of ALT, AST, and indirect bilirubin (IBIL), while the hydrogen-rich water combination group had no difference in liver function during treatment, possibly due to it. antioxidant activity, suggesting that it is a promising protective agent for ameliorating mFOLFOX6-induced liver injury
Most of the adverse effects of ionizing radiation on normal cells are caused by hydroxyl radicals. Combining radiation therapy with some forms of hydrogen may help alleviate these side effects (89). In fact, several studies have found that hydrogen can protect cells and mice from radiation (48, 90).
Tested in a rat skin injury model using a 44 Gy electron beam, the hydrogen-rich water-treated group had a higher proportion of SOD activity and less MDA and IL-6 in the injured tissue compared to the control group and distilled water. water group. In addition, hydrogen-rich water shortens the healing time and improves the healing rate of skin lesions (48).
Gastrointestinal toxicity is a common side effect induced by radiotherapy, which impairs the life quality of cancer patients (91). As shown in Xiao et al.’s study in mice model, hydrogen-water administration via oral gavage increased the survival rate and body weight of mice which were exposed to total abdominal irradiation, accompanied with an improvement in gastrointestinal tract function and the epithelial integrity of the small intestine. Further microarray analysis revealed that hydrogen-water treatment up-regulated miR-1968-5p, which then up-regulated its target myeloid differentiation primary response gene 88 (MyD88, a mediator in immunopathology, and gut microbiota dynamics of certain intestinal diseases involving toll-like receptors 9) expression in the small intestine after total abdominal irradiation (92).
Gastrointestinal toxicity is a common side effect of radiation therapy and affects the quality of life of cancer patients (91). As reported in the study by Xiao et al. It was demonstrated in a mouse model that administration of hydrogen water by oral gavage increased survival and body weight in mice exposed to whole abdominal irradiation, accompanied by improvements in gastrointestinal function and intestinal epithelial integrity. Further microarray analysis revealed that hydrogen water treatment up-regulated miR-1968-5p and then mutated its primary myeloid differentiation response gene 88 (MyD88, a mediator of immunopathology, and gut microbiota dynamics in certain intestinal diseases). Charges and recipients 9) in the small intestine after whole abdominal irradiation (92).
Hydrogen Gas Acts Synergistically With Thermal Therapy
A recent study found that hydrogen can enhance the effects of photothermal therapy. Zhao et al. Hydrated Pd nanocrystals (named PdH0.2) were designed as multifunctional hydrogen carriers for tumor-directed delivery (due to 30 nm cubic Pd nanocrystals) and controlled release of bioreductive hydrogen (due to lattice-bound hydrogen). PD). As shown in this study, hydrogen evolution can be modulated by the power and duration of near-infrared (NIR) irradiation. Treatment with PdH0.2 nanocrystals plus NIR irradiation resulted in higher initial ROS loss in cancer cells, and the subsequent ROS rebound was also much higher than in normal cells, resulting in more apoptosis and severe inhibition of mitochondrial metabolism in cancer cells, but abnormal cells. The combination of PdH0.2 nanocrystals and NIR irradiation greatly enhanced the anticancer efficacy of hyperthermia and achieved a synergistic anticancer effect. The in vivo safety assessment showed that the injection dose of 10 mg kg-1 PdH0.2 nanocrystals did not cause death, and there were no changes in many blood indicators, and no damage to liver and kidney function. In a 4T1 mouse breast cancer tumor model and a B16-F10 melanoma model, the combined treatment of PdH0.2 nanocrystals and NIR irradiation showed synergistic anticancer effects with significant tumor suppressive effects compared with hyperthermia. Meanwhile, the combination group showed no significant damage to the heart, liver, spleen, lung, and kidney, indicating appropriate tissue safety and tolerability (52).
Hydrogen Gas Suppresses Tumor Formation
Reader. Drinking hydrogen-rich water has been reported to ameliorate renal injury induced by iron nitrilotriacetate (Fe-NTA) in rats, as evidenced by decreased serum creatinine and BUN levels. Hydrogen-rich water inhibited Fe-NTA-induced oxidative stress by reducing lipid peroxidation ONOO-, inhibiting the activities of NADPH oxidase and xanthine oxidase, up-regulating antioxidant catalase, and restoring renal mitochondrial function. Therefore, hydrogen treatment significantly attenuated Fe-NTA-induced inflammatory cytokines such as NF-κB, IL-6, and monocyte chemoattractant protein 1. More importantly, drinking hydrogen-rich water inhibited the expression of several cancer-related proteins, including vascular endothelial growth factor, transcription-3-phosphorylation signal converter and activator, and proliferating nuclear antigen in rats, thereby reducing renal incidence of cancer. Cell carcinomas and their inhibition lead to tumor growth. This work demonstrates that hydrogen-rich water is a promising regimen to mitigate Fe-NTA-induced renal injury and suppress early tumor events.
Nonalcoholic steatohepatitis (NASH), caused by oxidative stress from various stimuli, is one of the causes of liver cancer (94, 95). In a mouse model, administration of hydrogen-rich water decreased hepatic cholesterol and peroxisome proliferator-activated receptor-alpha (PPARα) expression and increased hepatic antioxidant activity compared with control and pioglitazone-treated groups role (96). Hydrogen-rich water showed potent inhibitory effects on inflammatory cytokines TNF-α and IL-6, oxidative stress and apoptosis biomarkers. As shown in the NASH-related hepatocarcinogenesis model, the hydrogen-rich water-treated group had a lower tumor incidence and smaller tumor volume than the control and pioglitazone-treated groups. The above results suggest that hydrogen-rich water has the potential to protect the liver and treat liver cancer (96).
Hydrogen Gas Suppresses Tumor Growth
In addition to being used as an adjuvant therapy, hydrogen can also inhibit the growth of tumors and tumor cells.
As reported in the study by Wang et al. As demonstrated on lung cancer cell lines A549 and H1975 cells, hydrogen gas inhibits cell proliferation, migration and invasion and induces significant apoptosis, such as CCK-8, wound healing, transwell assays and flow cytometry tests. Hydrogen prevents the cell cycle in the G2/M phase of both cell lines by inhibiting the expression of several cell cycle regulatory proteins, including cyclin D1, CDK4, and CDK6. Chromosome 3 (SMC3), a complex required for chromosome condensation in the cell cycle (97), is inhibited by hydrogen through ubiquitination. Importantly, an in vivo study showed that hydrogen treatment significantly inhibited tumor growth, as well as the expression of Ki-67, VEGF and SMC3. These data suggest that hydrogen could be used as a novel approach to the treatment of lung cancer (98).
Due to its physicochemical properties, the use of hydrogen in hospitals, medical institutions and laboratories is severely restricted. Reader. A solidified hydrogen-absorbing (H2) silica was designed to stably release molecular hydrogen into the cell culture medium. H2-silicic acid may inhibit cell viability in human esophageal squamous cell carcinoma (KYSE-70) in a concentration-dependent manner, whereas it requires higher doses to inhibit normal human esophageal epithelial cells (HEEpiCs), indicating its selectivity . This effect was further confirmed by apoptosis and cell migration assays in these two cell lines. Mechanistic studies suggest that H2-silicic acid exerts its anticancer effects by inducing H2O2 accumulation, cell cycle arrest, and mitochondrial pathway-mediated induction of apoptosis (72).
Hydrogen has recently been found to inhibit cancer stem cells (CSCs). Hydrogen reduces colony formation and spheroidization in human ovarian cancer cell lines Hs38.T and PA-1 cells by inhibiting the proliferation marker Ki67, the stem cell marker CD34, and angiogenesis. Hydrogen treatment significantly inhibited the proliferation, invasion and migration of Hs38.T and PA-1 cells. More importantly, hydrogen inhalation significantly suppressed tumor volume, as shown in the Hs38.T xenograft BALB/c nude mouse model (99).
Another recent study also demonstrated the role of hydrogen in inhibiting glioblastoma (GBM), the most common malignant brain tumor. In vitro studies have shown that hydrogen gas inhibits several markers associated with stem formation, thereby inhibiting glioma cell spheroidization, cell migration, invasion, and colony formation. In a rat orthotopic glioma model, twice-daily inhalation of hydrogen gas (67%) for 1 hour significantly inhibited GBM growth and improved survival, suggesting that hydrogen gas may be a promising drug for GBM treatment (100).
Discussion
Hydrogen has been recognized as a medical gas with potential to treat cardiovascular disease, inflammatory disease, neurodegenerative disease and cancer (17, 60). As a hydroxyl radical and peroxynitrite scavenger, due to its anti-inflammatory effect, hydrogen can prevent/mitigate the side effects caused by chemotherapy and radiotherapy without affecting its anticancer potential (as shown in Table 1 and Figure 1). Hydrogen can also act alone or synergistically with other therapies to inhibit tumor growth by inducing apoptosis, inhibiting CSCs-related and cell cycle-related factors, etc. (summarized in Table 1).TABLE 1FIGURE 1What’s more, in most research papers, hydrogen is safe for cancer cells and some selectivity for normal cells, which is critical for clinical trials. A clinical study is underway in China to study the role of hydrogen in cancer recovery.
So far, several methods of administration have proven available and convenient, including inhalation, drinking hydrogen-dissolved water, injecting hydrogen-saturated saline, and performing a hydrogen bath. Hydrogen-rich water is non-toxic, inexpensive, easy to administer, and can easily diffuse into tissues and cells that cross the blood-brain barrier, suggesting its potential in the treatment of brain tumors. More well-structured and sufficiently secure wearables are needed.
References
1. De Bels D, Corazza F, Germonpre P, Balestra C. The normobaric oxygen paradox: a novel way to administer oxygen as an adjuvant treatment for cancer? Med Hypotheses. (2011) 76:467–70. 10.1016/j.mehy.2010.11.022 [PubMed] [CrossRef] [Google Scholar]2. Oliveira C, Benfeito S, Fernandes C, Cagide F, Silva T, Borges F. NO and HNO donors, nitrones, and nitroxides: past, present, and future. Med Res Rev. (2018) 38:1159–87. 10.1002/med.21461 [PubMed] [CrossRef] [Google Scholar]3. Vitek L, Gbelcova H, Muchova L, Vanova K, Zelenka J, Konickova R, et al. . Antiproliferative effects of carbon monoxide on pancreatic cancer. Dig Liver Dis. (2014) 46:369–75. 10.1016/j.dld.2013.12.007 [PubMed] [CrossRef] [Google Scholar]4. Flannigan KL, Wallace JL. Hydrogen sulfide-based anti-inflammatory and chemopreventive therapies: an experimental approach. Curr Pharm Des. (2015) 21:3012–22. 10.2174/1381612821666150514105413 [PubMed] [CrossRef] [Google Scholar]5. Li Z, Huang Y, Du J, Liu AD, Tang C, Qi Y, et al. . Endogenous sulfur dioxide inhibits vascular calcification in association with the TGF-beta/Smad signaling pathway. Int J Mol Sci. (2016) 17:266. 10.3390/ijms17030266 [PMC free article] [PubMed] [CrossRef] [Google Scholar]6. Jin H, Liu AD, Holmberg L, Zhao M, Chen S, Yang J, et al. . The role of sulfur dioxide in the regulation of mitochondrion-related cardiomyocyte apoptosis in rats with isopropylarterenol-induced myocardial injury. Int J Mol Sci. (2013) 14:10465–82. 10.3390/ijms140510465 [PMC free article] [PubMed] [CrossRef] [Google Scholar]7. Jiroutova P, Oklestkova J, Strnad M. Crosstalk between brassinosteroids and ethylene during plant growth and under abiotic stress conditions. Int J Mol Sci. (2018) 19:3283. 10.3390/ijms19103283 [PMC free article] [PubMed] [CrossRef] [Google Scholar]8. Paardekooper LM, van den Bogaart G, Kox M, Dingjan I, Neerincx AH, Bendix MB, et al. . Ethylene, an early marker of systemic inflammation in humans. Sci Rep. (2017) 7:6889. 10.1038/s41598-017-05930-9 [PMC free article] [PubMed] [CrossRef] [Google Scholar]9. Cui Q, Yang Y, Ji N, Wang JQ, Ren L, Yang DH, et al. Gaseous signaling molecules and their application in resistant cancer treatment: from invisible to visible. Future Med Chem. (2019) 11:323–6. 10.4155/fmc-2018-0403 [PubMed] [CrossRef] [Google Scholar]10. Huang Z, Fu J, Zhang Y. Nitric oxide donor-based cancer therapy: advances and prospects. J Med Chem. (2017) 60:7617–35. 10.1021/acs.jmedchem.6b01672 [PubMed] [CrossRef] [Google Scholar]11. Ma Y, Yan Z, Deng X, Guo J, Hu J, Yu Y, et al. . Anticancer effect of exogenous hydrogen sulfide in cisplatinresistant A549/DDP cells. Oncol Rep. (2018) 39:2969–77. 10.3892/or.2018.6362 [PubMed] [CrossRef] [Google Scholar]12. Zheng DW, Li B, Li CX, Xu L, Fan JX, Lei Q, et al. . Photocatalyzing CO2 to CO for enhanced cancer therapy. Adv Mater. (2017) 29:1703822. 10.1002/adma.201703822 [PubMed] [CrossRef] [Google Scholar]13. Chen J, Luo H, Liu Y, Zhang W, Li H, Luo T, et al. . Oxygen-self-produced nanoplatform for relieving hypoxia and breaking resistance to sonodynamic treatment of pancreatic cancer. Acs Nano. (2017) 11:12849–62. 10.1021/acsnano.7b08225 [PubMed] [CrossRef] [Google Scholar]14. Stuehr DJ, Vasquez-Vivar J. Nitric oxide synthases-from genes to function. Nitric Oxide. (2017) 63:29. 10.1016/j.niox.2017.01.005 [PMC free article] [PubMed] [CrossRef] [Google Scholar]15. Cao X, Ding L, Xie ZZ, Yang Y, Whiteman M, Moore PK, et al. . A review of hydrogen sulfide synthesis, metabolism, and measurement: is modulation of hydrogen sulfide a novel therapeutic for cancer? Antioxid Redox Signal. (2018) 31:1–38. 10.1089/ars.2017.7058 [PMC free article] [PubMed] [CrossRef] [Google Scholar]16. Zhai X, Chen X, Ohta S, Sun X. Review and prospect of the biomedical effects of hydrogen. Med Gas Res. (2014) 4:19. 10.1186/s13618-014-0019-6 [PMC free article] [PubMed] [CrossRef] [Google Scholar]17. Ostojic SM. Molecular hydrogen: an inert gas turns clinically effective. Ann Med. (2015) 47:301–4. 10.3109/07853890.2015.1034765 [PubMed] [CrossRef] [Google Scholar]18. LeBaron TW, Laher I, Kura B, Slezak J. Hydrogen gas: from clinical medicine to an emerging ergogenic molecule for sports athletes. Can J Physiol Pharmacol. (2019) 10:1–11. 10.1139/cjpp-2019-0067 [PubMed] [CrossRef] [Google Scholar]19. Guan P, Sun ZM, Luo LF, Zhao YS, Yang SC, Yu FY, et al. . Hydrogen gas alleviates chronic intermittent hypoxia-induced renal injury through reducing iron overload. Molecules. (2019) 24: 24:E1184. 10.3390/molecules24061184 [PMC free article] [PubMed] [CrossRef] [Google Scholar]20. Sakai D, Hirooka Y, Kawashima H, Ohno E, Ishikawa T, Suhara H, et al. . Increase in breath hydrogen concentration was correlated with the main pancreatic duct stenosis. J Breath Res. (2018) 12:36004. 10.1088/1752-7163/aaaf77 [PubMed] [CrossRef] [Google Scholar]21. Smith NW, Shorten PR, Altermann EH, Roy NC, McNabb WC. Hydrogen cross-feeders of the human gastrointestinal tract. Gut Microbes. (2018) 10:1–9. 10.1080/19490976.2018.1546522 [PMC free article] [PubMed] [CrossRef] [Google Scholar]22. Fukuda K, Asoh S, Ishikawa M, Yamamoto Y, Ohsawa I, Ohta S. Inhalation of hydrogen gas suppresses hepatic injury caused by ischemia/reperfusion through reducing oxidative stress. Biochem Biophys Res Commun. (2007) 361:670–4. 10.1016/j.bbrc.2007.07.088 [PubMed] [CrossRef] [Google Scholar]23. Liu C, Kurokawa R, Fujino M, Hirano S, Sato B, Li XK. Estimation of the hydrogen concentration in rat tissue using an airtight tube following the administration of hydrogen via various routes. Sci Rep. (2014) 4:5485. 10.1038/srep05485 [PMC free article] [PubMed] [CrossRef] [Google Scholar]24. Yamamoto R, Homma K, Suzuki S, Sano M, Sasaki J. Hydrogen gas distribution in organs after inhalation: real-time monitoring of tissue hydrogen concentration in rat. Sci Rep. (2019) 9:1255. 10.1038/s41598-018-38180-4 [PMC free article] [PubMed] [CrossRef] [Google Scholar]25. Dole M, Wilson FR, Fife WP. Hyperbaric hydrogen therapy: a possible treatment for cancer. Science. (1975) 190:152–4. 10.1126/science.1166304 [PubMed] [CrossRef] [Google Scholar]26. Ohsawa I, Ishikawa M, Takahashi K, Watanabe M, Nishimaki K, Yamagata K, et al. . Hydrogen acts as a therapeutic antioxidant by selectively reducing cytotoxic oxygen radicals. Nat Med. (2007) 13:688–94. 10.1038/nm1577 [PubMed] [CrossRef] [Google Scholar]27. Ostojic SM. Inadequate production of H2 by gut microbiota and Parkinson disease. Trends Endocrinol Metab. (2018) 29:286–8. 10.1016/j.tem.2018.02.006 [PubMed] [CrossRef] [Google Scholar]28. Hirayama M, Ito M, Minato T, Yoritaka A, LeBaron TW, Ohno K. Inhalation of hydrogen gas elevates urinary 8-hydroxy-2′-deoxyguanine in Parkinson’s disease. Med Gas Res. (2018) 8:144–9. 10.4103/2045-9912.248264 [PMC free article] [PubMed] [CrossRef] [Google Scholar]29. Meng J, Yu P, Jiang H, Yuan T, Liu N, Tong J, et al. . Molecular hydrogen decelerates rheumatoid arthritis progression through inhibition of oxidative stress. Am J Transl Res. (2016) 8:4472–7. [PMC free article] [PubMed] [Google Scholar]30. Shao A, Wu H, Hong Y, Tu S, Sun X, Wu Q, et al. . Hydrogen-rich saline attenuated subarachnoid hemorrhage-induced early brain injury in rats by suppressing inflammatory response: possible involvement of NF-kappaB pathway and NLRP3 inflammasome. Mol Neurobiol. (2016) 53:3462–76. 10.1007/s12035-015-9242-y [PubMed] [CrossRef] [Google Scholar]31. Gao Y, Yang H, Chi J, Xu Q, Zhao L, Yang W, et al. . Hydrogen gas attenuates myocardial ischemia reperfusion injury independent of postconditioning in rats by attenuating endoplasmic reticulum stress-induced autophagy. Cell Physiol Biochem. (2017) 43:1503–4. 10.1159/000481974 [PubMed] [CrossRef] [Google Scholar]32. Dozen M, Enosawa S, Tada Y, Hirasawa K. Inhibition of hepatic ischemic reperfusion injury using saline exposed to electron discharge in a rat model. Cell Med. (2013) 5:83–7. 10.3727/215517913X666486 [PMC free article] [PubMed] [CrossRef] [Google Scholar]33. Fan M, Xu X, He X, Chen L, Qian L, Liu J, et al. . Protective effects of hydrogen-rich saline against erectile dysfunction in a streptozotocin induced diabetic rat model. J Urol. (2013) 190:350–6. 10.1016/j.juro.2012.12.001 [PubMed] [CrossRef] [Google Scholar]34. Zhang X, Liu J, Jin K, Xu H, Wang C, Zhang Z, et al. . Subcutaneous injection of hydrogen gas is a novel effective treatment for type 2 diabetes. J Diabetes Investig. (2018) 9:83–90. 10.1111/jdi.12674 [PMC free article] [PubMed] [CrossRef] [Google Scholar]35. Tamura T, Hayashida K, Sano M, Suzuki M, Shibusawa T, Yoshizawa J, et al. . Feasibility and safety of hydrogen gas inhalation for post-cardiac arrest syndrome- first-in-human pilot study. Circ J. (2016) 80:1870–3. 10.1253/circj.CJ-16-0127 [PubMed] [CrossRef] [Google Scholar]36. Ge L, Yang M, Yang NN, Yin XX, Song WG. Molecular hydrogen: a preventive and therapeutic medical gas for various diseases. Oncotarget. (2017) 8:102653–73. 10.18632/oncotarget.21130 [PMC free article] [PubMed] [CrossRef] [Google Scholar]37. Ray PD, Huang BW, Tsuji Y. Reactive oxygen species (ROS) homeostasis and redox regulation in cellular signaling. Cell Signal. (2012) 24:981–90. 10.1016/j.cellsig.2012.01.008 [PMC free article] [PubMed] [CrossRef] [Google Scholar]38. Kumari S, Badana AK, G MM, G S, Malla R. Reactive oxygen species: a key constituent in cancer survival. Biomark Insights. (2018) 13:91914689. 10.1177/1177271918755391 [PMC free article] [PubMed] [CrossRef] [Google Scholar]39. Nita M, Grzybowski A. The role of the reactive oxygen species and oxidative stress in the pathomechanism of the age-related ocular diseases and other pathologies of the anterior and posterior eye segments in adults. Oxid Med Cell Longev. (2016) 2016:3164734. 10.1155/2016/3164734 [PMC free article] [PubMed] [CrossRef] [Google Scholar]40. Pelicano H, Carney D, Huang P. ROS stress in cancer cells and therapeutic implications. Drug Resist Updat. (2004) 7:97–110. 10.1016/j.drup.2004.01.004 [PubMed] [CrossRef] [Google Scholar]41. Liou GY, Storz P. Reactive oxygen species in cancer. Free Radic Res. (2010) 44:479–96. 10.3109/10715761003667554 [PMC free article] [PubMed] [CrossRef] [Google Scholar]42. Cui Q, Wang JQ, Assaraf YG, Ren L, Gupta P, Wei L, et al. . Modulating ROS to overcome multidrug resistance in cancer. Drug Resist Updat. (2018) 41:1–25. 10.1016/j.drup.2018.11.001 [PubMed] [CrossRef] [Google Scholar]43. Zhao Y, Hu X, Liu Y, Dong S, Wen Z, He W, et al. . ROS signaling under metabolic stress: cross-talk between AMPK and AKT pathway. Mol Cancer. (2017) 16:79. 10.1186/s12943-017-0648-1 [PMC free article] [PubMed] [CrossRef] [Google Scholar]44. Zha J, Chen F, Dong H, Shi P, Yao Y, Zhang Y, et al. . Disulfiram targeting lymphoid malignant cell lines via ROS-JNK activation as well as Nrf2 and NF-kB pathway inhibition. J Transl Med. (2014) 12:163. 10.1186/1479-5876-12-163 [PMC free article] [PubMed] [CrossRef] [Google Scholar]45. Gorrini C, Harris IS, Mak TW. Modulation of oxidative stress as an anticancer strategy. Nat Rev Drug Discov. (2013) 12:931–47. 10.1038/nrd4002 [PubMed] [CrossRef] [Google Scholar]46. Yu J, Yu Q, Liu Y, Zhang R, Xue L. Hydrogen gas alleviates oxygen toxicity by reducing hydroxyl radical levels in PC12 cells. PLoS ONE. (2017) 12:e173645. 10.1371/journal.pone.0173645 [PMC free article] [PubMed] [CrossRef] [Google Scholar]47. Li Y, Li Q, Chen H, Wang T, Liu L, Wang G, et al. . Hydrogen gas alleviates the intestinal injury caused by severe sepsis in mice by increasing the expression of heme oxygenase-1. Shock. (2015) 44:90–8. 10.1097/SHK.0000000000000382 [PubMed] [CrossRef] [Google Scholar]48. Zhou P, Lin B, Wang P, Pan T, Wang S, Chen W, et al. . The healing effect of hydrogen-rich water on acute radiation-induced skin injury in rats. J Radiat Res. (2019) 60:17–22. 10.1093/jrr/rry074 [PMC free article] [PubMed] [CrossRef] [Google Scholar]49. Iketani M, Ohshiro J, Urushibara T, Takahashi M, Arai T, Kawaguchi H, et al. . Preadministration of hydrogen-rich water protects against lipopolysaccharide-induced sepsis and attenuates liver injury. Shock. (2017) 48:85–93. 10.1097/SHK.0000000000000810 [PubMed] [CrossRef] [Google Scholar]50. Dong A, Yu Y, Wang Y, Li C, Chen H, Bian Y, et al. . Protective effects of hydrogen gas against sepsis-induced acute lung injury via regulation of mitochondrial function and dynamics. Int Immunopharmacol. (2018) 65:366–72. 10.1016/j.intimp.2018.10.012 [PubMed] [CrossRef] [Google Scholar]51. Yang Q, Ji G, Pan R, Zhao Y, Yan P. Protective effect of hydrogen-rich water on liver function of colorectal cancer patients treated with mFOLFOX6 chemotherapy. Mol Clin Oncol. (2017) 7:891–6. 10.3892/mco.2017.1409 [PMC free article] [PubMed] [CrossRef] [Google Scholar]52. Zhao P, Jin Z, Chen Q, Yang T, Chen D, Meng J, et al. . Local generation of hydrogen for enhanced photothermal therapy. Nat Commun. (2018) 9:4241. 10.1038/s41467-018-06630-2 [PMC free article] [PubMed] [CrossRef] [Google Scholar]53. Antonioli L, Blandizzi C, Pacher P, Hasko G. Immunity, inflammation and cancer: a leading role for adenosine. Nat Rev Cancer. (2013) 13:842–57. 10.1038/nrc3613 [PubMed] [CrossRef] [Google Scholar]54. Dermond O, Ruegg C. Inhibition of tumor angiogenesis by non-steroidal anti-inflammatory drugs: emerging mechanisms and therapeutic perspectives. Drug Resist Updat. (2001) 4:314–21. 10.1054/drup.2001.0219 [PubMed] [CrossRef] [Google Scholar]55. Shakola F, Suri P, Ruggiu M. Splicing regulation of pro-inflammatory cytokines and chemokines: at the interface of the neuroendocrine and immune systems. Biomolecules. (2015) 5:2073–100. 10.3390/biom5032073 [PMC free article] [PubMed] [CrossRef] [Google Scholar]56. Bottazzi B, Riboli E, Mantovani A. Aging, inflammation, and cancer. Semin Immunol. (2018) 40:74–82. 10.1016/j.smim.2018.10.011 [PubMed] [CrossRef] [Google Scholar]57. Zitvogel L, Pietrocola F, Kroemer G. Nutrition, inflammation, and cancer. Nat Immunol. (2017) 18:843–50. 10.1038/ni.3754 [PubMed] [CrossRef] [Google Scholar]58. Liu K, Lu X, Zhu YS, Le N, Kim H, Poh CF. Plasma-derived inflammatory proteins predict oral squamous cell carcinoma. Front Oncol. (2018) 8:585. 10.3389/fonc.2018.00585 [PMC free article] [PubMed] [CrossRef] [Google Scholar]59. Mager LF, Wasmer MH, Rau TT, Krebs P. Cytokine-induced modulation of colorectal cancer. Front Oncol. (2016) 6:96. 10.3389/fonc.2016.00096 [PMC free article] [PubMed] [CrossRef] [Google Scholar]60. Ning K, Liu WW, Huang JL, Lu HT, Sun XJ. Effects of hydrogen on polarization of macrophages and microglia in a stroke model. Med Gas Res. (2018) 8:154–9. 10.4103/2045-9912.248266 [PMC free article] [PubMed] [CrossRef] [Google Scholar]61. Zhang N, Deng C, Zhang X, Zhang J, Bai C. Inhalation of hydrogen gas attenuates airway inflammation and oxidative stress in allergic asthmatic mice. Asthma Res Pract. (2018) 4:3. 10.1186/s40733-018-0040-y [PMC free article] [PubMed] [CrossRef] [Google Scholar]62. Wardill HR, Mander KA, Van Sebille YZ, Gibson RJ, Logan RM, Bowen JM, et al. . Cytokine-mediated blood brain barrier disruption as a conduit for cancer/chemotherapy-associated neurotoxicity and cognitive dysfunction. Int J Cancer. (2016) 139:2635–45. 10.1002/ijc.30252 [PubMed] [CrossRef] [Google Scholar]63. Cheung YT, Ng T, Shwe M, Ho HK, Foo KM, Cham MT, et al. . Association of proinflammatory cytokines and chemotherapy-associated cognitive impairment in breast cancer patients: a multi-centered, prospective, cohort study. Ann Oncol. (2015) 26:1446–51. 10.1093/annonc/mdv206 [PMC free article] [PubMed] [CrossRef] [Google Scholar]64. Vyas D, Laput G, Vyas AK. Chemotherapy-enhanced inflammation may lead to the failure of therapy and metastasis. Onco Targets Ther. (2014) 7:1015–23. 10.2147/OTT.S60114 [PMC free article] [PubMed] [CrossRef] [Google Scholar]65. Padoan A, Plebani M, Basso D. Inflammation and pancreatic cancer: focus on metabolism, cytokines, and immunity. Int J Mol Sci. (2019) 20:E676. 10.3390/ijms20030676 [PMC free article] [PubMed] [CrossRef] [Google Scholar]66. Li FY, Zhu SX, Wang ZP, Wang H, Zhao Y, Chen GP. Consumption of hydrogen-rich water protects against ferric nitrilotriacetate-induced nephrotoxicity and early tumor promotional events in rats. Food Chem Toxicol. (2013) 61:248–54. 10.1016/j.fct.2013.10.004 [PubMed] [CrossRef] [Google Scholar]67. Huang D, Ichikawa K. Drug discovery targeting apoptosis. Nat Rev Drug Discov. (2008) 7:1041. 10.1038/nrd2765 [PubMed] [CrossRef] [Google Scholar]68. Pfeffer CM, Singh A. Apoptosis: a target for anticancer therapy. Int J Mol Sci. (2018) 19:E448. 10.3390/ijms19020448 [PMC free article] [PubMed] [CrossRef] [Google Scholar]69. Qiao L, Wong BC. Targeting apoptosis as an approach for gastrointestinal cancer therapy. Drug Resist Updat. (2009) 12:55–64. 10.1016/j.drup.2009.02.002 [PubMed] [CrossRef] [Google Scholar]70. Kumar S. Caspase 2 in apoptosis, the DNA damage response and tumour suppression: enigma no more? Nat Rev Cancer. (2009) 9:897–903. 10.1038/nrc2745 [PubMed] [CrossRef] [Google Scholar]71. Gao Y, Yang H, Fan Y, Li L, Fang J, Yang W. Hydrogen-rich saline attenuates cardiac and hepatic injury in doxorubicin rat model by inhibiting inflammation and apoptosis. Mediators Inflamm. (2016) 2016:1320365. 10.1155/2016/1320365 [PMC free article] [PubMed] [CrossRef] [Google Scholar]72. Li Q, Tanaka Y, Miwa N. Influence of hydrogen-occluding-silica on migration and apoptosis in human esophageal cells in vitro. Med Gas Res. (2017) 7:76–85. 10.4103/2045-9912.208510 [PMC free article] [PubMed] [CrossRef] [Google Scholar]73. Wang FH, Shen L, Li J, Zhou ZW, Liang H, Zhang XT, et al. . The chinese society of clinical oncology (CSCO): clinical guidelines for the diagnosis and treatment of gastric cancer. Cancer Commun. (2019) 39:10. 10.1186/s40880-019-0349-9 [PMC free article] [PubMed] [CrossRef] [Google Scholar]74. Verheij M, Vens C, van Triest B. Novel therapeutics in combination with radiotherapy to improve cancer treatment: rationale, mechanisms of action and clinical perspective. Drug Resist Updat. (2010) 13:29–43. 10.1016/j.drup.2010.01.002 [PubMed] [CrossRef] [Google Scholar]75. Sun YJ, Hu YJ, Jin D, Li JW, Yu B. Health-related quality of life after treatment for malignant bone tumors: a follow-up study in China. Asian Pac J Cancer Prev. (2012) 13:3099–102. 10.7314/APJCP.2012.13.7.3099 [PubMed] [CrossRef] [Google Scholar]76. Susanne K, Michael F, Thomas S, Peter E, Andreas H. Predictors of fatigue in cancer patients: a longitudinal study. Support Care Cancer. (2019) 120:425–32. 10.1007/s00520-019-4660-4 [PubMed] [CrossRef] [Google Scholar]77. Razzaghdoust A, Mofid B, Peyghambarlou P. Predictors of chemotherapy-induced severe anemia in cancer patients receiving chemotherapy. Support Care Cancer. (2019). 10.1007/s00520-019-04780-7 [Epub ahead of print]. [PubMed] [CrossRef] [Google Scholar]78. Schumacker PT. Reactive oxygen species in cancer cells: live by the sword, die by the sword. Cancer Cell. (2006) 10:175–6. 10.1016/j.ccr.2006.08.015 [PubMed] [CrossRef] [Google Scholar]79. Inoue A, Saijo Y, Maemondo M, Gomi K, Tokue Y, Kimura Y, et al. . Severe acute interstitial pneumonia and gefitinib. Lancet. (2003) 361:137–9. 10.1016/S0140-6736(03)12190-3 [PubMed] [CrossRef] [Google Scholar]80. Terasaki Y, Suzuki T, Tonaki K, Terasaki M, Kuwahara N, Ohsiro J, et al. . Molecular hydrogen attenuates gefitinib-induced exacerbation of naphthalene-evoked acute lung injury through a reduction in oxidative stress and inflammation. Lab Invest. (2019) 99:793–806. 10.1038/s41374-019-0187-z [PubMed] [CrossRef] [Google Scholar]81. Luo W, Wen G, Yang L, Tang J, Wang J, Wang J, et al. . Dual-targeted and pH-sensitive doxorubicin prodrug-microbubble complex with ultrasound for tumor treatment. Theranostics. (2017) 7:452–65. 10.7150/thno.16677 [PMC free article] [PubMed] [CrossRef] [Google Scholar]82. Shen BY, Chen C, Xu YF, Shen JJ, Guo HM, Li HF, et al. . Is the combinational administration of doxorubicin and glutathione a reasonable proposal? Acta Pharmacol Sin. (2019) 40:699–709. 10.1038/s41401-018-0158-8 [PMC free article] [PubMed] [CrossRef] [Google Scholar]83. Matsushita T, Kusakabe Y, Kitamura A, Okada S, Murase K. Investigation of protective effect of hydrogen-rich water against cisplatin-induced nephrotoxicity in rats using blood oxygenation level-dependent magnetic resonance imaging. Jpn J Radiol. (2011) 29:503–12. 10.1007/s11604-011-0588-4 [PubMed] [CrossRef] [Google Scholar]84. Kitamura A, Kobayashi S, Matsushita T, Fujinawa H, Murase K. Experimental verification of protective effect of hydrogen-rich water against cisplatin-induced nephrotoxicity in rats using dynamic contrast-enhanced CT. Br J Radiol. (2010) 83:509–14. 10.1259/bjr/25604811 [PMC free article] [PubMed] [CrossRef] [Google Scholar]85. Nakashima-Kamimura N, Mori T, Ohsawa I, Asoh S, Ohta S. Molecular hydrogen alleviates nephrotoxicity induced by an anti-cancer drug cisplatin without compromising anti-tumor activity in mice. Cancer Chemother Pharmacol. (2009) 64:753–61. 10.1007/s00280-008-0924-2 [PubMed] [CrossRef] [Google Scholar]86. Meng X, Chen H, Wang G, Yu Y, Xie K. Hydrogen-rich saline attenuates chemotherapy-induced ovarian injury via regulation of oxidative stress. Exp Ther Med. (2015) 10:2277–82. 10.3892/etm.2015.2787 [PMC free article] [PubMed] [CrossRef] [Google Scholar]87. Marco MR, Zhou L, Patil S, Marcet JE, Varma MG, Oommen S, et al. . Consolidation mFOLFOX6 chemotherapy after chemoradiotherapy improves survival in patients with locally advanced rectal cancer: final results of a multicenter phase II trial. Dis Colon Rectum. (2018) 61:1146–55. 10.1097/DCR.0000000000001207 [PMC free article] [PubMed] [CrossRef] [Google Scholar]88. Horimatsu T, Nakayama N, Moriwaki T, Hirashima Y, Fujita M, Asayama M, et al. A phase II study of 5-fluorouracil/L-leucovorin/oxaliplatin. (mFOLFOX6) in Japanese patients with metastatic or unresectable small bowel adenocarcinoma. Int J Clin Oncol. (2017) 22:905–12. 10.1007/s10147-017-1138-6 [PMC free article] [PubMed] [CrossRef] [Google Scholar]89. Chuai Y, Zhao L, Ni J, Sun D, Cui J, Li B, et al. . A possible prevention strategy of radiation pneumonitis: combine radiotherapy with aerosol inhalation of hydrogen-rich solution. Med Sci Monit. (2011) 17:Y1–4. 10.12659/MSM.881698 [PMC free article] [PubMed] [CrossRef] [Google Scholar]90. Mei K, Zhao S, Qian L, Li B, Ni J, Cai J. Hydrogen protects rats from dermatitis caused by local radiation. J Dermatolog Treat. (2014) 25:182–8. 10.3109/09546634.2012.762639 [PubMed] [CrossRef] [Google Scholar]91. Rodriguez ML, Martin MM, Padellano LC, Palomo AM, Puebla YI. Gastrointestinal toxicity associated to radiation therapy. Clin Transl Oncol. (2010) 12:554–61. 10.1007/s12094-010-0553-1 [PubMed] [CrossRef] [Google Scholar]92. Xiao HW, Li Y, Luo D, Dong JL, Zhou LX, Zhao SY, et al. . Hydrogen-water ameliorates radiation-induced gastrointestinal toxicity via MyD88’s effects on the gut microbiota. Exp Mol Med. (2018) 50:e433. 10.1038/emm.2017.246 [PMC free article] [PubMed] [CrossRef] [Google Scholar]93. Kang KM, Kang YN, Choi IB, Gu Y, Kawamura T, Toyoda Y, et al. . Effects of drinking hydrogen-rich water on the quality of life of patients treated with radiotherapy for liver tumors. Med Gas Res. (2011) 1:11. 10.1186/2045-9912-1-11 [PMC free article] [PubMed] [CrossRef] [Google Scholar]94. Phan J, Ng V, Sheinbaum A, French S, Choi G, El KM, et al. . Hyperlipidemia and nonalcoholic steatohepatitis predispose to hepatocellular carcinoma development without cirrhosis. J Clin Gastroenterol. (2019) 53:309–13. 10.1097/MCG.0000000000001062 [PubMed] [CrossRef] [Google Scholar]95. Ma C, Zhang Q, Greten TF. Non-alcoholic fatty liver disease promotes hepatocellular carcinoma through direct and indirect effects on hepatocytes. Febs J. (2018) 285:752–62. 10.1111/febs.14209 [PubMed] [CrossRef] [Google Scholar]96. Kawai D, Takaki A, Nakatsuka A, Wada J, Tamaki N, Yasunaka T, et al. Hydrogen-rich water prevents progression of non-alcoholic steatohepatitis and accompanying hepatocarcinogenesis in mice. Hepatology. (2012) 56:912–21. 10.1002/hep.25782 [PubMed] [CrossRef] [Google Scholar]97. Kissebah AH, Sonnenberg GE, Myklebust J, Goldstein M, Broman K, James RG, et al. . Quantitative trait loci on chromosomes 3 and 17 influence phenotypes of the metabolic syndrome. Proc Natl Acad Sci USA. (2000) 97:14478–83. 10.1073/pnas.97.26.14478 [PMC free article] [PubMed] [CrossRef] [Google Scholar]98. Wang D, Wang L, Zhang Y, Zhao Y, Chen G. Hydrogen gas inhibits lung cancer progression through targeting SMC3. Biomed Pharmacother. (2018) 104:788–97. 10.1016/j.biopha.2018.05.055 [PubMed] [CrossRef] [Google Scholar]99. Shang L, Xie F, Li J, Zhang Y, Liu M, Zhao P, et al. Therapeutic potential of molecular hydrogen in ovarian cancer. Transl Cancer Res. (2018) 7:988–95. 10.21037/tcr.2018.07.09 [CrossRef] [Google Scholar]100. Liu MY, Xie F, Zhang Y, Wang TT, Ma SN, Zhao PX, et al. . Molecular hydrogen suppresses glioblastoma growth via inducing the glioma stem-like cell differentiation. Stem Cell Res Ther. (2019) 10:145. 10.1186/s13287-019-1241-x [PMC free article] [PubMed] [CrossRef] [Google Scholar]101. Zhang JY, Liu C, Zhou L, Qu K, Wang R, Tai MH, et al. . A review of hydrogen as a new medical therapy. Hepatogastroenterology. (2012) 59:1026–32. 10.5754/hge11883 [PubMed] [CrossRef] [Google Scholar]102. Ohta S. Recent progress toward hydrogen medicine: potential of molecular hydrogen for preventive and therapeutic applications. Curr Pharm Des. (2011) 17:2241–52. 10.2174/138161211797052664 [PMC free article] [PubMed] [CrossRef] [Google Scholar]103. Dixon BJ, Tang J, Zhang JH. The evolution of molecular hydrogen: a noteworthy potential therapy with clinical significance. Med Gas Res. (2013) 3:10. 10.1186/2045-9912-3-10 [PMC free article] [PubMed] [CrossRef] [Google Scholar]